Who ordered the doughnut: You or your gut bugs?
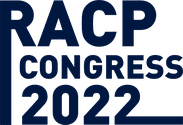
In 2022, Professor Wayne Cutfield was awarded the Howard Williams Medal for his outstanding contribution to improving the health of children and young people in Australia and Aotearoa New Zealand. The following is an edited version of Professor Cutfield’s oration – Who ordered the doughnut: You or your gut bugs? – which he delivered at RACP Congress 2022.
Professor Wayne Cutfield is professor of paediatric endocrinology at the Liggins Institute, and an expert on insulin sensitivity and action in children. He leads clinical research in three broad areas: (i) environmental influences early in life that affect childhood growth and metabolism in ways that could lead to chronic conditions in adult life; (ii) child obesity prevalence, causes and prevention; and (iii) the role of the gut microbiome in childhood disorders. A former director of the Liggins Institute, he is Director of the National Science Challenge: A Better Start. This is a cross-institution 10-year Government funded research program based at the institute.
Professor Cutfield commenced his address recognising past winners of the Howard Williams Medal and noting that the list read like a who’s who of leaders of child health research and care in Australia and New Zealand. He outlined the two parts of his presentation – early research work in the developmental origins field, and what he and his group are doing now in gut microbiome research. He pointed out that the title of his talk would become more evident later.
“The conventional dogma is that our risks of adult disease are due to two things, our genes and our lifestyle. If you have unfortunate genes and an awful lifestyle, then you are at risk of a range of communicable diseases. David Barker challenged that concept.”
Professor Cutfield expounded on how David Barker introduced the concept that early life events also influence our risk of later health and disease. His work was based in part on very intricate records kept by Margaret Burnside and other healthcare nurses in Hertfordshire and surrounding counties in England in the early 20th century.
David took that data and looked at the relationship between birth events, focusing largely on birth size and later adult diseases. He “found associations with reduced birth weight and diabetes, metabolic syndrome, chronic lung disease, heart disease, early mortality”. Barker then went on to show that with progressive reduction in birth weight, there is an increased risk of type-2 diabetes. That led him to propose the foetal origins hypothesis, that is, “the origins of adult disease begin in utero”. And he proposed that foetal malnutrition was a cause of small birth size and programmed later adult disease. At that time, he had no evidence that nutrition was a key player, and it wasn’t until later studies, notably the 2nd World War studies, the Dutch Winter Famine Study and the Leningrad Siege Study, that it was shown compromised nutrition was associated with later metabolic programming.
“I reasoned that if those of lower birth weight develop diabetes in later adult life, then there should be some link between these two periods.” In fact, that’s the case. “We studied 5 to 11-year-old children who were born at the more extreme end of the lower birth weight range, i.e. those born small for gestational age (birth weight <10th percentile). We measured insulin sensitivity using our modification of the minimal model which is a very complex and precise model that we use.”
Lower levels of insulin sensitivity (i.e. insulin resistance) is bad and the SGA children had about a 50 per cent reduction in sensitivity. This was the first-time evidence of metabolic programming in childhood, following Barker's work, had been shown. So, why do we care about insulin resistance if it isn’t diabetes? A study by Faccihini and colleagues took a group of healthy 40-year old’s and measured their insulin sensitivity, putting them into three groups, insulin sensitive, not so sensitive, and resistant groups. They followed them for up to 10 years to see what diseases they developed. In the insulin resistant group, they saw hypertension, cancer, heart disease, type 2 diabetes and strokes, whereas the insulin sensitive group remained healthy, without any of these major diseases occurring. So, insulin resistance is a major risk factor for a range of serious non-communicable diseases, not just diabetes.
“Rapid weight gain for any of us is a bad thing, but for this group the effects seem to be exaggerated in terms of risk of diabetes.”
Interestingly, regular exercise seems to have a more positive effect for this group than for normal subjects in terms of improving diabetes risk. That group is around 10 per cent of the population, as SGA defined. There are also groups of children who are exposed in foetal life to environmental adversity and are at risk of developmental programming, who were not thought to have these risks previously. These are common groups, including children born very prematurely. “By that I mean those born less than 32 weeks gestation. These children are similar to SGA children in that both groups are exposed to a compromised environment during the last trimester.”
The SGA children have suffered from utero placental insufficiency and sub-optimal nutrition. Despite the sophistication of neonatal intensive care, it cannot match in utero care, so it’s again a period of environmental compromise for those born very prematurely. Are there similarities between these groups? We went back and measured insulin sensitivity using our minimal model method and found a 50 per cent reduction in insulin sensitivity, which was similar to that of the SGA children we had studied. Previously, in terms of long-term risks of prematurity, the focus had all been on lung disease, cognitive function and vision, and the concept that there could be risks of later metabolic disease had never really been considered.
We then went about trying to understand what components of early nutrition or care could be contributing to metabolic programming. Dissecting out nutritional intake, which was all very carefully recorded in the neonatal intensive care notes, we looked at nutritional records hour by hour, day by day, week by week, for the first three months of life. We found that protein intake was lower than recommended by a number of international bodies, and that was offset by increased carbohydrate intake. And, in fact, increased carbohydrate intake was associated with an increased risk of obesity in mid-childhood. Since that study was published, neonatal intensive care has continued to evolve through the introduction of artificial surfactant, high-frequency ventilation and higher protein intake diets. Have things got better? Do they still suffer from development or programming? The answer is yes.
There is a reduction in sensitivity in the pre-term group, but not as severe as it was before these measures. Not 50 per cent, but more like a 25 to 30 per cent reduction. Changes in neo-natal intensive care have reduced this programming risk, but not ameliorated it. Professor Cutfield and colleagues have now extended this research to adults born preterm. These adults were markedly insulin resistant and also had greater amounts of body fat. Furthermore, they had greater amounts of abdominal fat, which conveys a higher risk of cardio-metabolic disease.
This was exaggerated in males who had an increase of 11 kilograms on average of predominantly abdominal fat. To see if the effects of prematurity could be transmitted to the next generation, they also studied their children who were born at term and completely normal and healthy. They too had increased body fat and increased abdominal fat. These prepubertal children had an additional 3 kilograms of fat when matched against controls, so they appear to have transgenerational programming towards a greater risk of abdominal fat and obesity. The possible cause of these programmed changes is discussed later.
Professor Cutfield then shifted his talk to another group who had adversity early, those born post-term (i.e. beyond 42 weeks gestation).
“In our initial post-term study, we had access to data from 500 16-year-old Swedish children who had intricate auxological data right through childhood. When we compared the post-term with term boys and girls, at the same height, the post-term boys were heavier than the term boys by about 11 kilograms, with a dramatic 47 per cent of the post-term boys obese at 16 years of age. Are there other metabolic parameters that are abnormal in the post-term group? We intricately studied an Auckland post-term group of prepubertal children and found a 50 per cent reduction in insulin sensitivity, higher night-time blood pressure based on 24-hour blood pressure monitoring, and a poor lipid profile. To date the post-term group appear to have the most extensive cardiometabolic abnormalities of any group we have studied.
“I have focused on children born SGA pre-term and post-term, however there are other common groups we have studied that were exposed to a suboptimal foetal environment. In large, for gestational age children, twins, children born to mothers with severe hyperemesis, or pre-eclampsia, there is a common phenotype. As there is progressive deviation away from an optimal in utero environment (e.g. born too early, too late, too small or too big), there are increased risks of long-term disease, and a risk of developmental programming.
“The common theme is the phenotype seems to be the same, and it is insulin resistance, excessive abdominal fat, high blood pressure and disordered lipids. These are all of the components of the metabolic syndrome commonly seen in adults.
“What could link these events over decades and how could you potentially get a transgenerational effect? Epigenetics has been proposed as that cause. There are slithers of data that would suggest that epigenetics through changes in DNA methylation and gene silencing may be an important player in these programme effects and could also lead to transgenerational changes.”
Let me finish the developmental programming part of my presentation by sharing data on the commonest group anyone could study, those who are first born!
“What about first borns? There is data that suggests that first borns are smarter and that that effect is environmental.”
A study found that first borns are smarter, but if the first born dies early in infancy, the second born is as smart as the first born would have been. And if the first born and second born die early, the third born is as smart as the first born should have been. That suggests that intelligence across a family is largely environmentally determined. However, we found first borns are taller as well.
In our first study we found that first-born children in mid-childhood are taller and the difference is about two to three centimetres taller than later born children. We adjusted for parents’ heights and stage of biological maturity (bone age). Looking at another very large cohort of sibling peers and adjusting for bone age, and biological maturation, we noted a sequence in which first borns are taller than second borns, who are in turn taller than third borns. When you look across a large population, first borns are taller. Of course, you will all know of exceptions, but across populations these findings are consistent.
Now, first borns have slightly lower birth weight, so have they had some degree of early nutritional compromise? Looking at the metabolic profiles of these children, as with the other cohorts, we looked into measuring insulin sensitivity and we found that first borns have reduced insulin sensitivity. Not as dramatically as other groups, but about a 25¬30 per cent reduction in insulin sensitivity. In addition, their daytime systolic and diastolic blood pressures measured by 24-hour ambulatory blood pressure were higher.
“Why is that? We adjusted for birth size just to be sure there wasn’t some sort of subtle SGA effect going on. It’s not clear what has caused those subtle metabolic programming abnormalities.”
Professor Cutfield suggested that it was possible that it was due to the dilation of the spiral arteries during pregnancy. In a second pregnancy, they’re already dilated, and they dilate further, so there’s greater blood flow in the second and subsequent pregnancies, so that may be a factor. The findings we’ve made about first borns, he noted, have been replicated by others.
Professor Cutfield then proceeded to speak about his fascination with the role of the gut microbiome in our health, wellbeing and behaviour, an exciting new field that has really only been explored over the past 15 years. He cited a landmark study where a germ-free mouse with no gut bacteria received gut microbiome from non-identical human twins, one twin obese, and the other not. What happened? The mice that received the microbiome from the obese twin became obese, whereas the mice that received the microbiome from the slim twin remained slim. What happened when they put the two together? Which phenotype win? We know that mice eat each other’s stools, enabling a simple microbiome treatment experiment. Interestingly, the thin phenotype won, both the obese and the slim mouse became slim.
Professor Cutfield then proceeded to studies looking at reduced gut microbiome diversity (dysbiosis), which is associated with a range of conditions, including obesity, type-2 diabetes, liver disease, inflammatory bowel disease, autism, Parkinson’s, allergic diseases like asthma, eczema, Alzheimer’s disease and many others. He went on to clarify that this association does not prove cause and effect and to do that you need to do microbiome treatment studies.
“Microbiome diversity is important, and it helps with resilience.” Now if gut bacteria can lead to obesity, or reverse obesity, how does that happen? The influence of the microbiome occurs at many different levels. Gut bacteria can provide an additional 200 kilocalories each day by breaking down non-digestible complex carbohydrates, and some of their products that are absorbed promote lipogenesis as well as inflammation in our bodies. A number of bacterial products are inflammatory and influence our immune system, and some of those products actually influence our appetite and behaviour.
“So, the title of my talk becomes a bit more evident. Who ordered the donut? Slightly facetious, but who was making the call? Did you make the call or did your gut microbiome signal your appetite centre to order the doughnut?” He noted, for example, that LPS increased intestinal permeability, which allowed bacterial products to get into the circulation. There are more than 30 or 40 bacterial products in our circulation, including molecules like short chain fatty acids which are proinflammatory. Short chain fatty acids are also thought to stimulate PYY, GLP-1 and other appetite and behaviour regulating products, influencing our eating behaviour, our cognition and impulsivity, our anxiety and our mood.
The most effective and convincing evidence that treating somebody with a healthy gut microbiome will make a difference comes from chronic C difficile infection, a debilitating disorder with a high mortality rate. Traditional therapies included vancomycin, which only had about a 30 per cent chance of success, so recurrent chronic C difficile was the norm. If you use a gut microbiome transfer, also called a faecal microbiome transfer or transplant, then you can effectively treat them and expect a 90 per cent cure rate, which is why it has become the treatment of choice for recurrent or persistent C difficile infection.
So, what does a microbiome transfer or faecal microbiome transfer involve? Early treatment approaches involve a healthy donor providing a fresh stool, which is put in a blender, and then a tube is inserted down into the small bowel or up into the bowel. “Sounds awful but for patients suffering from potentially life-threatening C difficile infection this treatment can be curative, thus it is an easy decision for patients to make.
“Given the exciting mouse studies and association studies in humans, it seemed the right time to progress to a microbiome treatment trial in obesity. Our gut bugs trial was the first trial in which gut microbiome treatment had been used to try and treat adolescent obesity.” The rationale was that if we took the gut microbiome from super healthy donors and gave it to severely obese teenagers, we could see if weight loss occurred. This was a double blind, randomised, placebo-controlled trial. We had 40 obese teen females and 40 obese teen males.
“We undertook assessment of recipients at baseline and throughout the 6 months post-treatment period. Each obese teenager received the microbiomes of four super donors to maximise microbiome diversity. Male donors to male recipients and female donors to female recipients. Donor screening was really rigorous, starting off with more than 100 potential donors to select the eight best. Donors had very extensive testing including body composition studies, metabolic studies, and microbiome studies. The participants were treated either with a placebo or the treatment, then we followed them up over six months and looked intricately across a wide range of outcomes as association studies suggest that the microbiome has a wide effect on health, well-being and behaviour.
“We developed a more acceptable way to deliver the microbiome treatment beyond the early draconian techniques of sigmoidoscopy and nasojejunal tube delivery of stool slurry. We established a double encapsulation technique. We took the bacteria out of a fresh donor stool, cleaned them up, put them in one capsule, and then inside a second capsule that could be swallowed and released into the bowel. This avoided the low pH of the stomach leading to bacterial destruction. We found that if we did that and quickly froze the capsules at minus 80 and then later thawed them, the encapsulated bacteria remained viable for more than three months.
“Unfortunately, we did not see the much-hoped weight loss at six weeks (primary outcome). There have been several other studies since, that have shown the same thing, no weight loss on follow-up after microbiome treatment in obese adults. Now why is that? If we go back to the previous study with the twins, what happens when you put the fat mouse and the thin mouse together and put them on a poor diet (a cafeteria diet), what wins? The thin mouse’s microbiome or the poor diet? The answer is the poor diet, which I’m sure was a problem in our teens. While we tried to recommend a healthy diet, when we watched the documentary on our study, we saw the teens eating lots of high fat high sugar food and takeaways. We still do not know if the combination of a calorie restriction diet and microbiome treatment could lead to greater weight loss than diet alone.”
Anecdotally, two of the participants at the end of the trial put themselves on a restricted diet. They had done it before and it did not work, but this time, they lost more than 10 kilos in six weeks. Professor Cutfield suggests the microbiome might be helpful in the treatment of obesity but only when added to calorie restriction, but that is yet to be tested.
What we did find is that metabolic syndrome in these obese teenagers basically resolved over the six-month period, and that there are very few treatments that reverse metabolic syndrome as effectively as this. While there wasn’t a reduction in total body fat, there was a reduction in abdominal fat right throughout the study, in both males and females, but particularly females.
Another interesting outcome was that, when we gave the microbiome treatment, it changed the obese teens’ microbiomes, and they remained changed at the six-month follow-up. “This single treatment, given over a couple of days to give them enough capsules, led to engraftment of a new microbiome that six months later was still there. Diversity in the donors was really key. Donor microbiome diversity increases the likelihood of successful engraftment. We also found that there was an increase [in] the prevotella to bacteroides ratio, both in females and males, and there’s some indirect evidence that may be made metabolically advantageous.”
Professor Cutfield concluded his presentation by thanking his team and talking about future research. “We’re expanding our metabolic syndrome studies because we want to try and find which bacteria might be particularly helpful.” This kind of treatment paradigm is clunky, labour intensive and expensive. “What we need to identify are either key bacteria or bacterial products that have clinical benefits and then grow those in culture. We’re also doing a double blind, randomised, placebo-controlled trial in autism looking to see if we can change some of the gut dysfunction, anxiety and other wellbeing parameters in those with autism. We are also doing a pilot study in anorexia nervosa, the opposite model to obesity. Those with anorexia nervosa have a very disrupted microbiome. Can a healthy microbiome assist weight gain during recovery from anorexia nervosa?”
In addition, he said they were developing both human gut and brain organoids in vitro that will enable them to look at the effects of bacterial products and bacteria, which will help their understanding of the role of the microbiome and which bacteria and bacterial products might be beneficial.